Cosmic whispers beckon from the distant past
Gravitational waves reveal the invisible universe
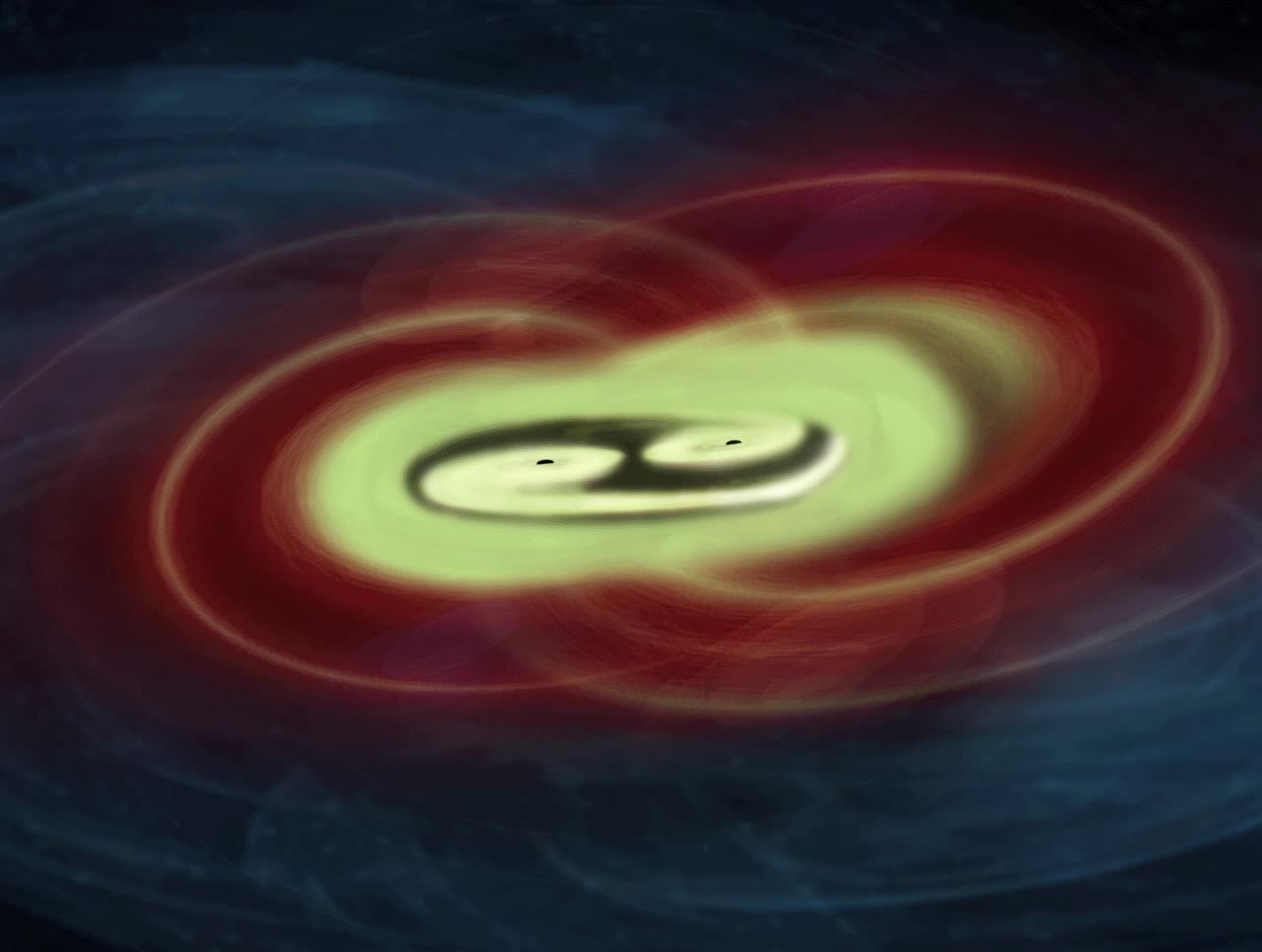
New Zealand is building a stake in two leading edge, multi-nation, astronomy research projects.
Astrophysicist Richard Easther confesses his profession is no different to others. He and colleagues like to know the goss. So, in the second half of September 2015, he heard from a very reputable source that the first much theorised, often-predicted, long-awaited gravitational wave had been detected. There was barely suppressed chatter and excitement in the world of astrophysics, Easther among them.
In his office, where he is today a Professor of Physics at Waipapa Taumata Rau, University of Auckland, he mimics the sound. “CCCHHHRRRRIIIPPP,” he goes, the chirp elongating and rising in tone. There you have it, the sound detected by a pair of gravitational wave detectors when two big black holes, each more than 30 times the mass of our sun, merge, spinning together in a last dance a billion light years away from the Earth.
When they slammed together, the pair of black holes generated enormous gravitational forces, briefly blazing with 50 times the energy of all the stars in the observable universe. From their merger came the gravitational wave now called GW150914.
Easther can do the math but, for the rest of us, gravitational waves are best described as wrinkles in spacetime, ephemeral distortions in gravitational fields that pass through the universe. Albert Einstein first predicted their existence and a billion-dollar investment in hyper precision engineering proved he was right.
The official announcement was made early in 2016, timed to honour Einstein’s brilliance a century prior. Three of the leading scientists involved Rainer Weiss, Barry Barish and Kip Thorne were awarded the Nobel Prize in physics the following year.
For Easther, an informed observer, detecting a gravitational wave was a big deal but more a matter of when not if. “It wasn’t like suddenly seeing a second sun or new planet. For me, as a physicist, but not working directly on that project, it felt more like physics had completed a long race.”
The finish line was confirmation that we had another way of ‘seeing’ the universe besides the electromagnetic spectrum, where telescopes use visible light and other electromagnetic waves to paint a picture of the universe.
The mathematics of gravitational waves had been sorted by the 1960s, he says. But figuring out how to listen to this message from space would take a little longer. The chirp of GW150914 was the achievement of the Laser Interferometer Gravitational Wave Observatory (LIGO), the definition of a fundamental science project. Funded by the US National Science Foundation and many partners around the world, LIGO comprises two installations, one in Hanford, Washington, the other in Livingstone, Louisiana.
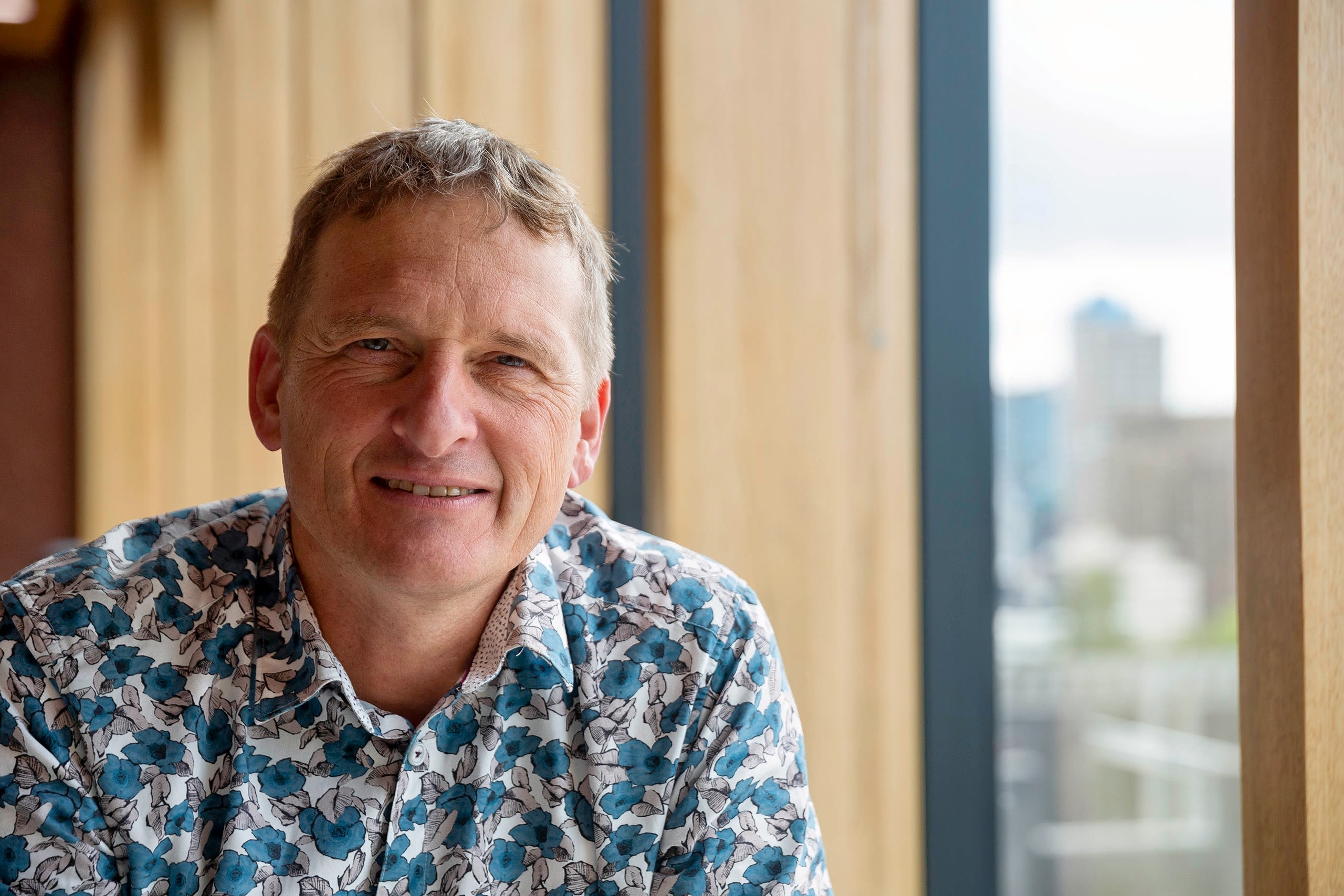
Easther says, “The arms of LIGO are 4km a side, (the structure is like a huge capital L) and what it is effectively able to do is measure the change in the length of the arms to a tolerance of about 1000th the width of the nucleus of an atom.”
The theory, now proven, is that as a gravitational wave passes through the array, it creates a tiny distortion in spacetime, stretching one arm, compressing the other. The two installations serve as a double check to ensure that this tiniest of phenomena is indeed a gravitational wave and not the rumble of a distant car.
The success of LIGO gave a big boost to an even more ambitious project from the European Space Agency and partner organisations, the LISA (Laser Interferometer Space Antenna) Consortium. LISA comprises three spacecraft each 2.5m km apart orbiting in a triangle formation about 150 million kilometres from the sun.
LISA’s three spacecraft form a space-based gravitational wave observatory able to detect gravitational waves to the level of 1 trillionth of a metre. If LIGO is like a stethoscope listening for a pulse, LISA will be a MRI scanner, able to discern shape and structure, where the patient is the universe.
LISA is science right at the edge of what’s possible and the questions it seeks to answer are fundamental: How did the universe begin? Why did it develop the way it has? What does this mean for the future?
No single country funds science at this scale. It takes a coalition, not only of the willing, but also those able to understand the wider value research in fundamental science offers. Easther says, “The benefits that come to countries pursuing this kind of thing, are huge and here we’re missing out on that.”
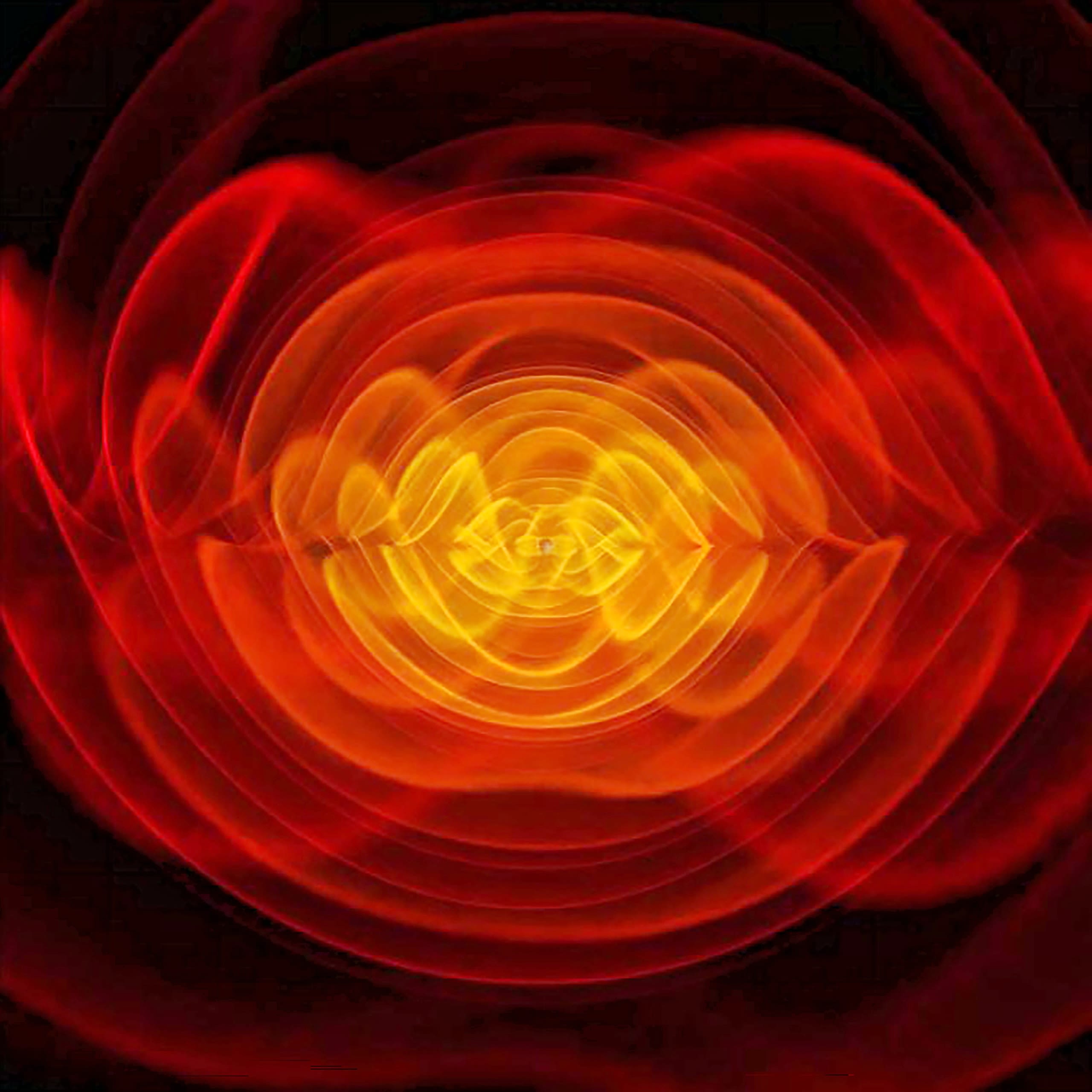
LISA: the Laser Interferometer Space Antenna consists of three sensors forming a triangle 2.5m km on each side in the same orbit as the Earth.
LISA: the Laser Interferometer Space Antenna consists of three sensors forming a triangle 2.5m km on each side in the same orbit as the Earth.
Big science needs leaps in innovation that create new technologies, materials and analytic techniques.
Big science needs leaps in innovation that create new technologies, materials and analytic techniques. New knowledge trickles down to everyday uses. Physics students master the higher mathematical and computational skills Big Tech covets. Former students and postdoctoral researchers of Easther can be found working at Amazon, Apple and RocketLab.
Easther felt strongly that New Zealand researchers needed to have a seat at the table. “I wanted to look for ways we could collaborate on big projects that were absolutely at the cutting edge of research.”
What could New Zealand contribute to LISA, one of the most ambitious big science projects in the world? It turns out that exploration of fundamental science is not the sole preserve of major economies.
Colleague Renate Meyer, Professor of Statistics at the University, following her own interests, had produced novel statistical techniques to interpret the data from gravitational wave detectors. (See The search for meaning in a universe of noise.)
With Marsden funding, Easther and Meyer built a network of researchers from across the country. Their full name is the New Zealand Astro Statistics and General Relativity Working Group, but are better referred to as New Zealand Gravity, bringing together collaborators from the Universities of Auckland, Victoria, Canterbury, Otago and Auckland University of Technology, to create the critical mass of expertise and experience to be accepted as a member of the International LISA consortium.
New Zealand Gravity, says Easther, can be the scaffold to support New Zealand researchers wanting to focus on aspects of fundamental science and creates a pathway for postdoctoral fellows to do their work here. If you want to know about cosmology and the evolution of stars, dark matter, gravitational waves and general relativity, talk to New Zealand Gravity.
LISA is much more sensitive to things that have to do with the overall structure of the universe. How did the Big Bang itself work?
For Easther, LISA offers the possibility to know more about how galaxies form and, with that, add to our understanding of the earliest shape of the universe. Gigantic black holes are found at the centre of galaxies and big galaxies like our Milky Way grow from the mergers of smaller galaxies. When galaxies merge their central black holes also eventually combine. LISA should shed light on how galaxies form by detecting the gravitational wave signals from the mergers of these 'supermassive' black holes.
Easther says, “The gravitational waves they produce are a low, low frequency if you want to think about it in musical terms. Because LISA is physically much bigger than LIGO, it will pick these up.”
While LIGO can detect the end of individual stars, LISA can map the gravitational waves emanating from the birth and growth of galaxies.
“LISA is much more sensitive to things that have to do with the overall structure of the universe. How did the Big Bang itself work? Those signals are things that I'm keen to look at. There are many ways the Big Bang might have happened. Some of those ways would have produced a bath of gravitational waves that filled the universe.”
This year the LISA consortium received the go-ahead to start developing the three space vehicles. The timeline sees a launch date in 2035. “By looking for gravitational waves we can test different theories of the universe. I’ve got a theory of the early universe I can work out whether it makes gravitational waves. If it does, then how and how big would they be?”
Until LIGO and LISA, our understanding of the universe has been formed almost entirely by what we can see through the electromagnetic spectrum, from visible light, x-rays, radio waves to infra-red and microwaves.
Each time we look to the skies, we see the past. The further we look the deeper in the past because the speed of light is a constant measure. By the time we see a distant star, the light it emits will have travelled millions and even billions of years to reach us. When we see our sun, it is always 8.3 light-minutes in the past.
The age of the universe has been calculated as 13.8 billion years. It is an undoubted triumph that today we can peer at the beginnings of the universe a mere 380,000 years after the Big Bang. Before this, the baby universe was a dense cloud of ionised plasma, so dense light could not pass. As the universe expanded and thinned, a flash of microwave energy, an echo from the Big Bang, known as the cosmic microwave background (CMB), was emitted, first detected in 1965.
LISA could open a window into the earliest moments of the Big Bang. “The universe is expanding and since the Big Bang, the minimum it has grown is 60 factors of 10. (1, followed by 60 zeroes.) But 45 of those 60 factors of ten happened in the first trillionth of a second of existence,” Easther says.
So, if more than half of the expansion of the universe happened in the first trillionth of a second and if, like Easther, you want to know about the crucible of energy and fundamental particles as the universe came into being, one approach is to be part of the team looking at the data LISA will gather.
If this sounds absurdly ambitious, think again. Easther says. In fewer than 50 years the leap in science, technology and mathematics has brought us far closer to understanding how the universe was born. “This is an unprecedented time in human history to be trying to figure out how the universe works. Why wouldn’t we want New Zealand to be a part of that?”
The search for meaning in a universe of noise
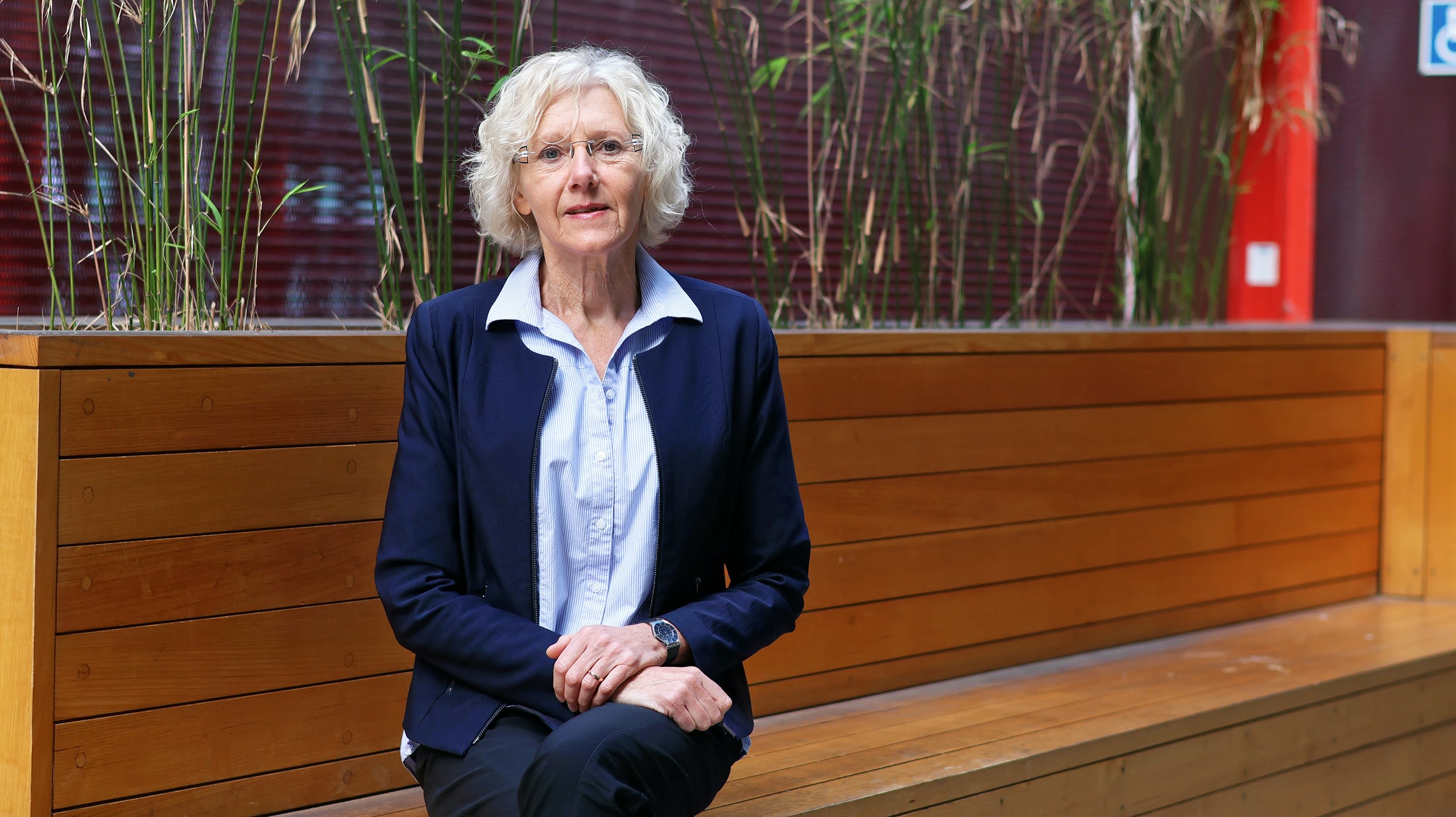
Renate Meyer and Nelson Christensen built the tools to extract insights from gravitational wave signals.
Professor of Statistics at the University of Auckland, Renate Meyer recalls it as one of those corridor conversations. Physicist Nelson Christensen, then also at the University, said, “Oh, we’ve got this problem where we have a lot of parameters”. This was her entry to gravitational waves, a field where her statistician’s brain led to a significant breakthrough.
Meyer says, “We worked together and simulated the gravitational wave signals we assumed that LIGO had detected. We were able to extract the parameters.”
As the physicist Christensen could mathematiclaly describe the astrophysics, the attributes like the size, orbit, velocity, shape and the electromagnetic and gravitational forces of phenomena like blackholes and neutron stars. These became the 'parameters' Meyer's statistics could distil from gravitational wave signals.
Their paper Markov Chain Monte Carlo Methods for Bayesian Gravitational Radiation Data Analysis was published on 8 September 1998 in Physical Review, the pre-eminent journal for physics research.
Their work resulted in a genuine breakthrough, with many papers to follow. The methods they outlined remain the foundation to understanding the nature of gravitational waves. And, not least, their significant contribution to one of the most ambitious big science projects undertaken came from New Zealand.
It helps to go back to the late 1990s when gravitational waves existed only in the realm of theory and high-level mathematics. Even before they were finally detected, those in the field faced a problem. It was all very well to detect gravitational waves, but the bigger game was to wring meaning from their signals.
The first ‘chirrup’ detected in 2015 lasted only seconds. That signal, and any signal, is a package of data. Meyer and Christensen’s breakthrough made it possible to decode the signal. Meyer says: “Gravitational waves are embedded in the noise of the universe. These tiny waves come to us amidst ‘noise’ that is much, much larger.
" So, if you observe this little wiggle, next you want to say something about what causes it. Are they two black holes that orbit around each other? And, if so, how big are these two black holes? And where are they? That’s the challenge. How do you extract all that from this little wiggle?”
It fell to Meyer and statistical analysis of those tiny perturbations in the gravitational wave signal to say with confidence that the tiny wriggle of that first ‘chirrup’ was the echo of two black holes, 20 to 30 times the mass of our sun, colliding at a significant proportion of the speed of light, hundreds of light years away.
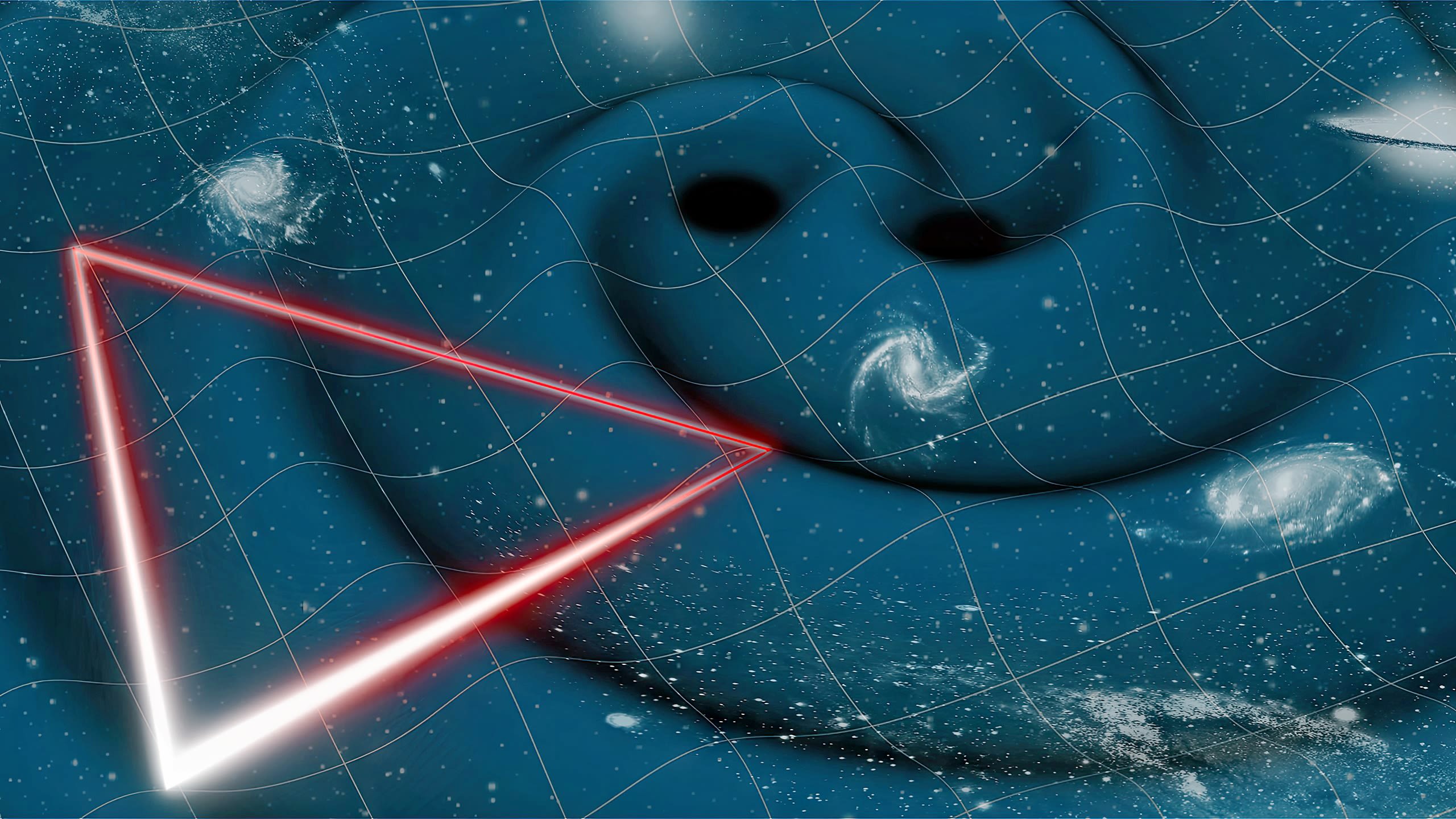
“With LISA we’re dealing with hundreds of millions of data points.”
Think of the task as extracting an high-resolution, full-colour image from a blurry shot taken by a pinhole camera.
It helps to break down the concepts in the title of Meyer and Christiansen’s influential paper. A Markov chain, named for Russian mathematician Andrey Markov, is a way to predict the future where the only thing that’s important is the present ‘state’. A state could be anything. For example, it could be the rooms in a house. A person in a room decides to move to the next room based on the room they’re in, rather than how they got to the room. Each time the person moves to another room, this generates a probability. Together each move becomes a link in a Markov chain of probabilities.
The Monte Carlo technique is like spinning a roulette wheel. Each spin produces a new result. Traditionally the casino always wins, but in this instance, the Monte Carlo technique allows statisticians to generate many possible arrangements of the ‘parameters’.
On a roulette wheel this would be the odds of a specific number and whether red or black. For a gravitational wave, it would be the probable range of astrophysical phenomena. The trick is then to focus the simulations of what might be there hundreds of light years away to show what is actually happening.
Unsurprisingly, this takes an inordinate amount of computer power and Meyer’s work would be impossible without access to the New Zealand eScience Infrastructure’s super computers and Marsden funding that has enabled her to work with a wider group of researchers.
The statistical breakthroughs to turn the LIGO gravitational wave signals into a new picture of the universe face an exponentially harder challenge once LISA, the space based gravitational wave observatory launches.
The chirrups from LIGO last about a second and carry perhaps 4,000 data points. Meyer says: “With LISA we’re dealing with hundreds of millions of data points.”
As one of the few in the world to apply statistics to gravitational wave detection and, at the time, the only statistician in New Zealand, Meyer's work was often solitary.
She now has a group of peers, post-doctoral researchers and PhD students who meet each Friday. When you speak a rare language, it must be a comfort to talk with familiar voices.
A glimpse into the infinite future
At the peak of Cerro Pachón, a mountain in northern Chile, work is underway on a telescope that will offer a unique view of the night sky.
Due to begin operations in 2025, the Vera C Rubin Observatory, funded by the American National Science Foundation and the US Department of Energy, will start the Legacy Survey of Space and Time.
With a light sensor the size of a small car, and panoramic lenses and over the span of three to four nights, the Rubin Observatory will be able to capture a view of the entire night sky. It will continue to do this for the next ten years. From this bounty of new imagery, the observatory is expected to capture the finer detail of about 17 billion stars and 20 billion galaxies.
Alongside LISA, Easther is part of a New Zealand wide group building a collaboration with this project. Rubin will detect objects ranging from large rocks inside our own solar system that may hit Earth, through to supernovae – exploding stars visible halfway across the universe
He says, “One of the things we will do is use the images to map out the position of galaxies in the sky. By constructing more intricate and detailed maps, more of the structure of the universe will be revealed."
Easther says: “From those patterns, we can begin to understand not just how old the universe is but begin to tell the story of how it began, why it looks the way it does today, and what it will look like into the infinite future.”
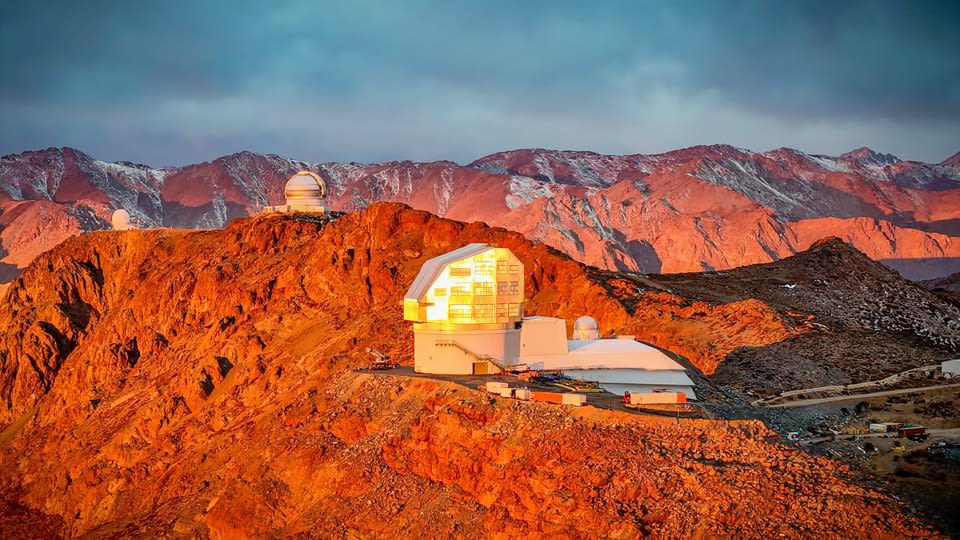
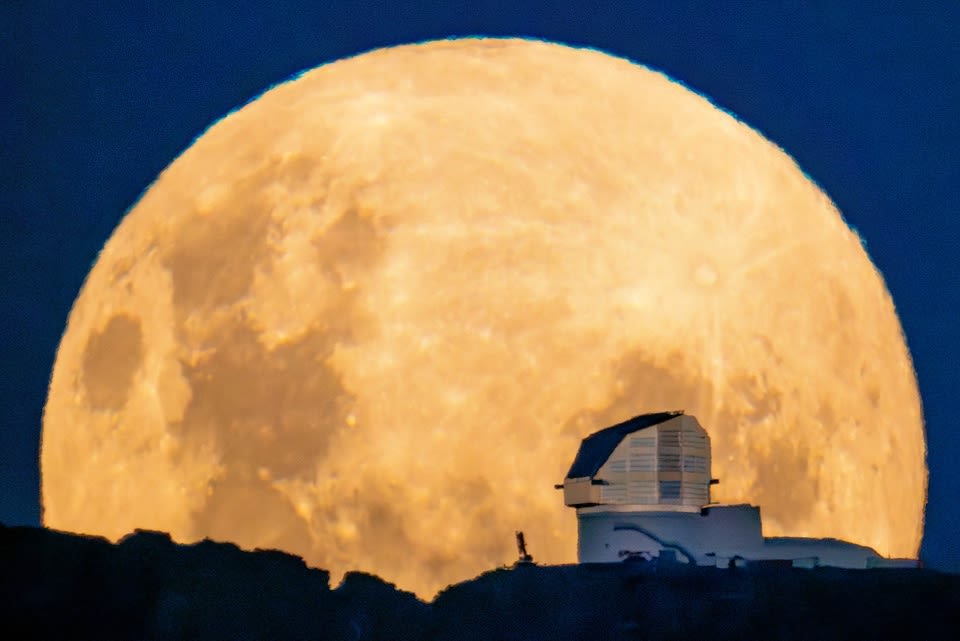
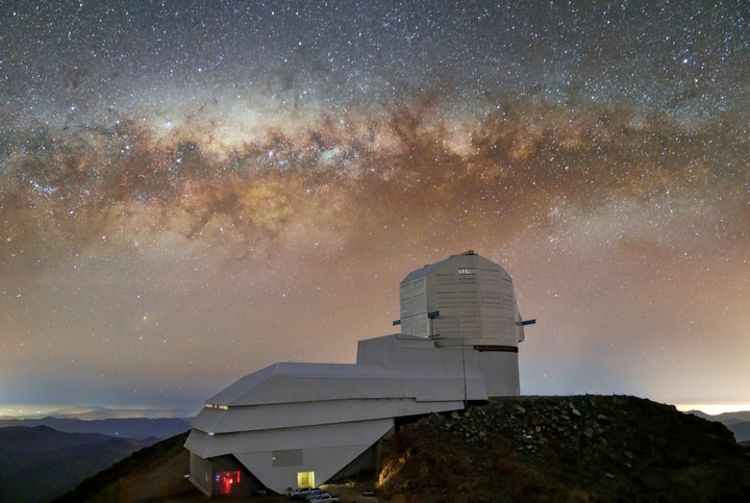
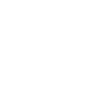